All published articles of this journal are available on ScienceDirect.
Invasive Electrical Impedance Tomography for Blood Vessel Detection
Abstract
We present a novel method for localization of large blood vessels using a bioimpedance based needle positioning system on an array of ten monopolar needle electrodes. The purpose of the study is to develop a portable, low cost tool for rapid vascular access for cooling and controlled reperfusion of cardiac arrest patients. Preliminary results show that localization of blood vessels is feasible with this method, but larger studies are necessary to improve the technology.
1. INTRODUCTION
Despite investments in research, training, equipment and infrastructure, survival from unexpected cardiac arrest has been virtually unchanged over the past couple of decades. On average, the survival rate is approximately 5 % in the USA/Europe, but can even be as low as 2 % in the bigger cities or above 20 % in those cities with the best implementation of science and education [1,2].
Time from cardiac arrest until cardiopulmonary resuscitation and defibrillation is one factor influencing survival. Another factor affecting survival is reperfusion injury. Cell death does not only take place as a result of ischemia, but even more as a function of reperfusion. Given the situation of sudden cardiac arrest, there is now evidence to suggest that most of the cell death and subsequent irreversible organ damage takes place as a consequence of uncontrolled reperfusion [3]. With controlled reperfusion, where there is full control of flow, pressure and composition of the reperfusing fluid, cell and organ damage can be substantially reduced.
A third factor that can improve survival is induced hypothermia. We know that therapeutic hypothermia is beneficial after cardiac arrest, and that intra-arrest cooling is beneficial both with respect to defibrillation success and survival to discharge from hospital. Cooling also seems to slow down the speed of cell death caused by reperfusion after cardiac arrest [4].
Hence, both rapid intra-arrest cooling and controlled reperfusion are interventions which may substantially extend the time window of opportunity after cardiac arrest, drowning or trauma. A prerequisite for achieving this, however, is the development of new methods for obtaining vascular access in a secure and effective manner.
In this paper we present a novel method for localization of large blood vessels using a bioimpedance based needle positioning system reported earlier [5]. The main purpose is to gain vascular access for cooling and controlled reperfusion of the patient. An array of ten needles is plunged into the tissue and then stepped out again while electrical impedance spectra are recorded at each step for all ten needles. Different tissue types, such as blood, muscle or fat, will have characteristic impedance spectra and we can hence continuously determine what sort of tissue the needle tips are in. The basic idea is then to use needles located in large blood vessels as guides for cannulae to obtain vascular access.
2. MATERIALS AND METHODS
We have earlier reported on a new method for impedance-based tissue discrimination for needle guidance [5]. This method is based on sending a small sensing current through the needle for measurement of electrical impedance in a three-electrode setup. Due to the small cross section and hence high current density on the tip of the needle, the measured impedance will be totally dominated by the volume within a radius of approximately 2 mm from the needle tip.
In this study we constructed an array of 10 Teca Monopolar Needle Electrodes (37mm x 28G) from Viasys Healthcare, mounted in a plastic block (Fig. 1). The shaft of the needles is electrically insulated, leaving only the sharp tip in galvanic contact with the tissue. The center-to-center distance between the needles was 3 mm.
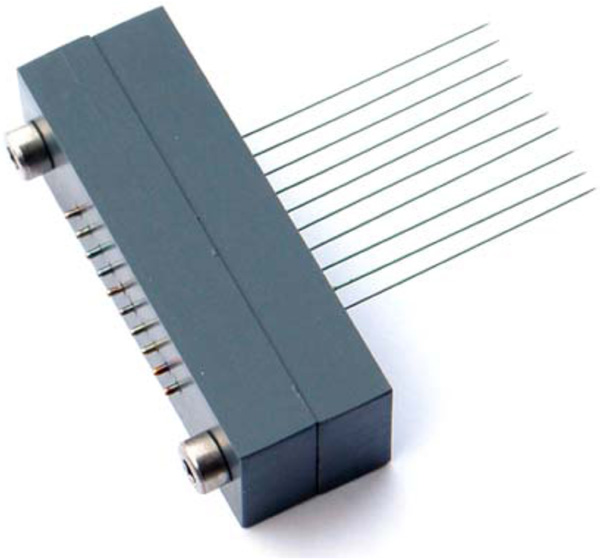
Array of ten Viasys Healthcare Teca needles used for impedance measurements to produce a 2D invasive impedance tomogram.
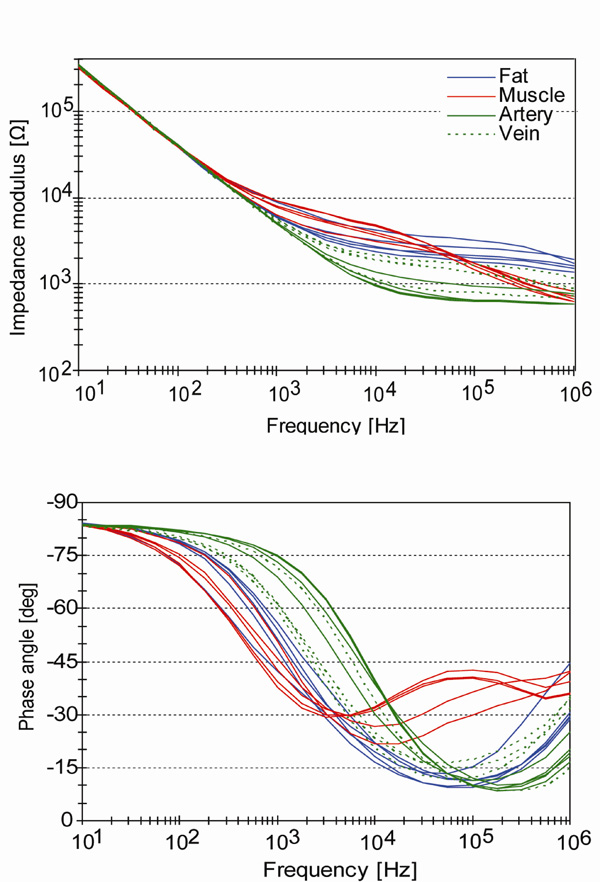
Impedance spectra of Teca needles in different tissue types in an anesthetized pig.
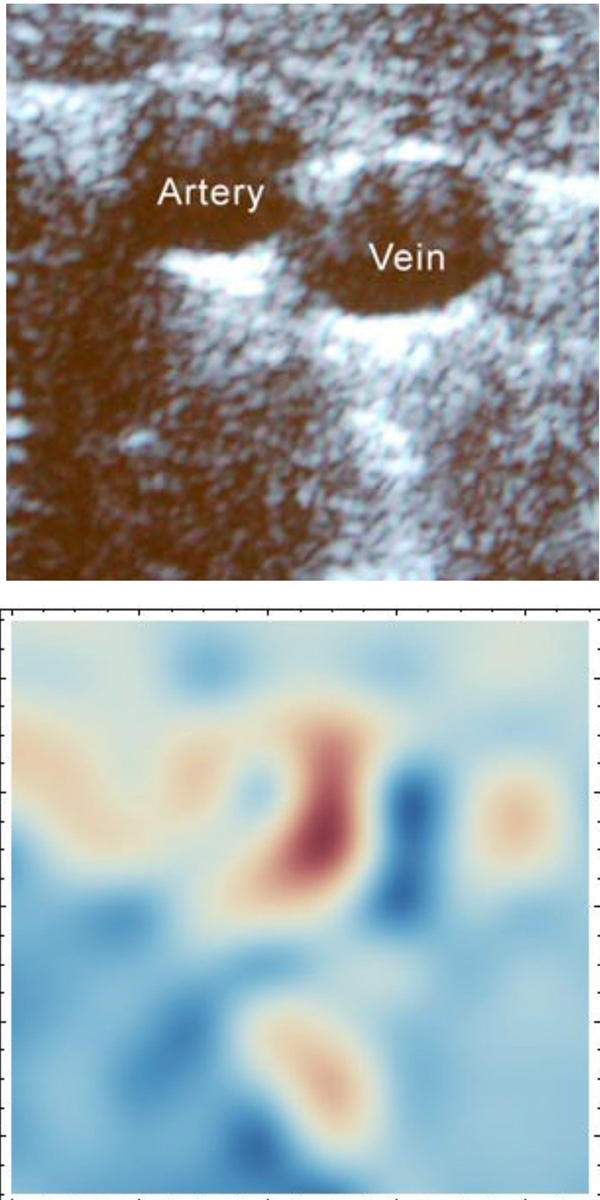
Ultrasound image and plot of impedance phase angle at 316 kHz from approximately the same femoral area of an anesthetized pig, showing an artery and a vein. The phase angle ranges from 3.9 deg (red) to 49.7 deg (blue).
The study was approved by the Institutional Animal Care and Use Committee. We used a 55 kg Norwegian Landrace pig. The animal was supplied by the Centre for Comparative Medicine, Rikshospitalet University Hospital, Oslo, Norway. The femoral artery and vein of the anesthetized pig were located by ultrasound. The array was then pushed into the same region across the artery and vein until the plastic block was in contact with the pig skin (approx. 37 mm depth). The needles were connected through a Keithley 7001/7011S multiplexer to a Solartron 1260+1294 impedance analyzer. Each needle then served as a measuring electrode in a three-electrode system, where the reference and counter current electrodes were Ambu “Blue Sensor Q” ECG electrodes on the pig skin. After the insertion of the needles, the electrical impedance of each needle tip was measured successively with the Solartron system, using an applied voltage of 30 mV rms and frequencies between 10 Hz and 1 MHz. Then a 3 mm Plexiglas spacer was inserted between the plastic block and the pig skin, so that the needles were pulled out 3 mm. A new frequency scan was then performed on all needles, a new spacer introduced, then another frequency scan, etc. In this way a ten by ten matrix of impedance curves was attained.
As a first attempt of an invasive impedance tomography plot, we simply plotted the phase angle at 316 kHz for each pixel in the matrix. The chosen frequency is also in accordance with the findings of Salazar et al. [6]. The plotting was done in Mathematica 7 (Wolfram Research) using a “ListDensityPlot” with third order polynomial interpolation and a red-to-blue color map.
3. RESULTS
Fig. (2) shows an example of single needle measurements in different tissue types carried out in an earlier study by Kalvøy et al. [5]. Frequencies up to at least 10 kHz are dominated by electrode polarization impedance and do not represent intrinsic biomaterial electrical properties. However, it is obvious that also the electrode impedance is tissue dependent, as pointed out by Kalvøy et al. [5] so the low frequency part of the spectra are also of interest for tissue discrimination.
Fig. (3) shows the plot of the phase angle at 316 kHz together with a section of the ultrasound image from approximately the same area.
4. DISCUSSION
The phase angle plot in Fig. (3) shows resemblance to the ultrasound image. The artery comes out quite clear, but with a somewhat oblate shape. The vein has a lower phase angle leading to a lighter red color. As shown in Fig. (2), venous blood is more similar to fat in this frequency range so the light red areas in the lower and left part of the plot are more difficult to interpret. It could be bleeding (the acquisition of the data for the image took more than an hour) or fatty tissue. Since this plot is based on only one single parameter (phase angle at 316 kHz), a better separation of venous blood from other tissue types will probably be achieved when using a full multivariate model.
It is important to note, however, that the measurements presented in this paper were acquired during full perfusion and not during cardiac arrest. Hence, further studies must be performed to investigate how lack of circulation will influence the measured data.
5. CONCLUSION
These preliminary result show that needle array measurements may prove valuable as a tool to guide needles into particular tissue types, such as blood vessels. Producing an image is not a necessary step in such an application, but it represents a convenient way of assessing the method. To our knowledge, this is the first time a percutaneous, invasive, electrical impedance tomography image is presented. The quality of the image, and hence the method, will probably be greatly improved when multivariate methods are used for classification of the tissue. Furthermore, when the proper multivariate model is developed, the instrumentation can probably be simplified to using only a few simultaneous measuring frequencies for time domain or frequency domain spectroscopy, thus significantly reducing the acquisition time down to a few seconds.