All published articles of this journal are available on ScienceDirect.
Immune Checkpoint Inhibitors in Therapeutics and their Adverse Effect Profile: A Review
Abstract
Objective
Immune Checkpoint Inhibitors (ICIs) have transformed the field of oncology by improving the functional ability of the immune system to combat malignancies. This review investigates the mechanisms of ICIs, their adverse effects, resistance mechanisms, and the role of Artificial Intelligence (AI) And Machine Learning (ML) in predicting treatment outcomes.
Methods and Materials
A literature search was conducted using PubMed, Google Scholar, and Web of Science to identify pertinent studies, clinical trials, and review articles. The study concentrated on seven ICIs that have been approved and are designed to target the PD-1, PD-L1, and CTLA-4 pathways. The data were derived from clinical guidelines and expert opinions.
Results
ICIs have illustrated efficacy in a variety of malignancies, such as renal cell carcinoma, non-small cell lung cancer, and melanoma. Their utilization, whether as monotherapy or in conjunction with chemotherapy, radiotherapy, or targeted therapies, has substantially enhanced survival. Nevertheless, the management of Immune-Related Adverse Events (irAEs) that affect multiple organ systems is imperative. In certain patients, the efficacy of ICI is also restricted by resistance mechanisms. AI/ML-driven models demonstrate potential for anticipating patient responses, optimizing treatment strategies, and reducing toxicity risks.
Conclusion
ICIs have revolutionized cancer therapy; however, there are still obstacles in predicting responses and managing adverse effects. This review emphasizes the innovative use of AI/ML to improve the precision and safety of ICI. Nevertheless, additional research is required due to the absence of reliable predictive biomarkers and the variability of patient responses. In order to enhance treatment outcomes and reduce toxicity, future research should enhance AI-driven models and incorporate multi-omics approaches.
1. INTRODUCTION
The immune system, a robust defense mechanism that protects individuals against infections and cancer cells, can sometimes become altered and fail to properly identify and eliminate checkpoints and improve immune system activity for tumor suppression or eradication in order to solve this issue [1]. ICIs are frequently used in conjunction with other forms of therapy to increase the body's defenses against tumor cells and raise treatment efficacy overall [2]. By employing unique techniques to target cancer cells, the two primary classes of Immune Checkpoint Inhibitors (ICIs), namely CTLA-4 and PD-1 and PD-L1 inhibitors, have demonstrated encouraging outcomes recently [3].
Although these developments have been made, ICIs are not universally efficacious, and both primary and acquired resistance continue to be significant obstacles [4]. In some patients, the limited responses that are observed are a result of the immunosuppressive tumor microenvironment, insufficient neoantigen presentation, or inadequate infiltration of tumor-reactive CD8+ T cells in many tumors. Furthermore, sustained exposure to ICIs can lead to Immune-related Adverse Events (irAEs), which can affect a variety of organs, including the lungs, liver, and endocrine glands [5]. The efficacy of treatment is being improved by the exploration of combination strategies that involve ICIs with chemotherapy, targeted therapy, or radiotherapy in order to surmount these limitations. Additionally, predictive biomarkers, including PD-L1 expression, Tumor Mutational Burden (TMB), and microsatellite instability (MSI), are currently being examined to enhance patient selection and treatment personalization [6]. Although ICIs have revolutionized cancer treatment, it is imperative to conduct continuous research to address their limitations, enhance patient stratification, and create innovative therapeutic combinations [7]. Therefore, in the present review, we summarize a) the mechanism of action of the ICIs, b) various adverse effects of ICIs, c) case studies and resistance mechanisms, and d) the role of AI and ML in predicting the pros and cons of the ICI.
1.1. Search Strategy
We accessed PubMed, Google Scholar, and Web of Science to include high-quality, interdisciplinary studies.
1.2. Search Terms and Boolean Operators
We used a detailed search strategy incorporating both keywords and controlled vocabulary (e.g., MeSH terms) to ensure a comprehensive and targeted literature search. Keywords included: “immune checkpoint inhibitors,” “ICIs,” “adverse effects,” “immune-related adverse events,” “PD-1,” “PD-L1,” “CTLA-4,” “resistance mechanisms,” “clinical trials,” “machine learning,” and “artificial intelligence.” Boolean logic was applied to refine the search, using combinations such as: “immune checkpoint inhibitors” AND (“adverse effects” OR “immune-related adverse events”), and “PD-1” OR “PD-L1” OR “CTLA-4” AND (“clinical trial” OR “case study” OR “review”). This approach ensured both sensitivity and specificity in identifying relevant studies.
1.3. Search Limits, Filters, and Inclusion and Exclusion Criteria
Search limits and filters included only the articles published in English.
We applied specific inclusion and exclusion criteria to ensure the selection of high-quality and relevant studies. Inclusion criteria encompassed studies focusing on the application of Immune Checkpoint Inhibitors (ICIs) in oncology, their associated adverse events, resistance mechanisms, or the integration of artificial intelligence and machine learning in predicting treatment outcomes. We included clinical trials, meta-analyses, systematic reviews, and case reports involving human subjects, provided they were published as peer-reviewed full-text articles. Exclusion criteria were applied to filter out articles unrelated to ICIs or their adverse effects, non-English publications, non-peer-reviewed materials, such as editorials or opinion pieces lacking primary data, and animal-only studies unless they had direct translational relevance to human applications. Studies presenting duplicative data already covered in other sources were also excluded.
1.4. Selection Process and Data Extraction and Analysis
We followed a structured and transparent selection process to identify eligible studies. We screened the titles and abstracts to assess relevance, with studies clearly not meeting the inclusion criteria being excluded at this stage. Subsequently, full-text versions of the remaining articles were retrieved and thoroughly reviewed against the predefined inclusion and exclusion criteria to confirm their eligibility. For data extraction and analysis, we used a standardized data extraction form to ensure consistency and reliability. This form captured essential information, such as study design, sample size, patient demographics, types of ICIs used, outcome measures including efficacy, adverse events, and resistance mechanisms, as well as specific notes on the use of artificial intelligence and machine learning methodologies, where applicable.
1.4.1. Mechanism of Action of Immune Checkpoint Inhibitors (ICIs)
A protein termed CTLA-4, which functions as an immune system regulator, is present in T-lymphocytes [8]. ICIs enable T-cells to become more active and efficiently destroy cancer cells by inhibiting CTLA-4. Furthermore, PD-1/PD-L1 inhibitors block the expression of the PD-L1 ligand in cancer cells [5, 9]. Because of this interference, T-cells are able to eradicate cancer cells, and the tumor is unable to elude the immune system. The over-expression of immunological checkpoints may impair the immune system's ability to identify and eliminate cancer cells. The development of CTLA-4 inhibition as a cancer immunotherapy strategy was made possible by the identification of CTLA-4's role in T-cell negative regulation. Humanized monoclonal antibodies were created to block the interaction between CTLA-4 and its ligand (B7) in cancer patients after preliminary animal experiments produced encouraging findings [10]. With an emphasis on effectively stimulating the immune system as a therapeutic target, this signaled a dramatic change in the field of cancer immunotherapy.
1.4.2. CTLA-4 Inhibitors
By interacting with its ligands CD80 and CD86, CTLA-4, a co-inhibitory member of the immunoglobulin superfamily, proves to be essential in preventing T cell activation [11]. CD28 binds these ligands as well, but CTLA-4 has a higher affinity and competes with CD28 for binding, which causes T cells to signal negatively [12]. CTLA-4 is effective in “the early phases of T-cell response in lymph nodes because the ligands are mainly produced on antigen-presenting cells [13]. T-cell proliferation is uncontrollably increased in the absence of CTLA-4 inhibitors. In models of breast, prostate, lymphoma, colon, and melanoma cancers, animal research has shown encouraging anti-tumor responses [14-18]. Human IgG1 monoclonal antibody (mAb) against CTLA-4, ipilimumab, was first approved in 2010 for the treatment of melanoma [19]. This treatment showed promise for long-term illness control, a persistent response that lasted longer than 2.5 years, and a notable increase in survival [20, 21].
1.4.3. Programmed Cell Death Protein-1 (PD-1) Inhibitors
In response to T-cell-mediated events, PD-1, another member of the immunoglobulin superfamily, is essential in suppressing the signaling that leads to programmed cell death [22]. As compared to CTLA-4, PD-1 is more broadly expressed and present in the tumor micro-environment (TME) across a range of immune cell types. T-cell suppression and eventual exhaustion result from an inhibitory signal that PD-1 sends when it attaches to its ligand, PD-L1 (B7-H1) [23-25]. The primary distinction between PD-1 and CTLA-4 is that the latter predominantly functions to restrict T-cell activity in peripheral tissues during later phases of tumor progression, whereas CTLA-4 regulates T-cell activity during the priming phase [26].
1.5. PD-L1 Inhibitors
PD-1 engages in interactions with its ligands, PD-L1 and PD-L2, which are expressed on normal dendritic cells, immune cells, and malignancies, in that order. Activated immune cells are suppressed by this interaction [27, 28]. Tumor cells can proliferate abnormally because they use the PD-1/PD-L1 pathway to weaken T-cell-mediated immunity. For this reason, immunotherapy targets PD-L1 effectively. Atemalizumab, Durvalumab, and Avelumab are the humanized IgG1 anti-PD-L1 monoclonal antibodies that have received FDA approval. Of them, atezolizumab was authorized in 2016 to treat urothelial carcinoma [29]. The mechanism of action, indication, dose, and year of approval of these drugs are given in Table 1.
1.5.1. Challenges Associated with Immune Checkpoint Inhibitors
Even though ICIs are revolutionizing immunotherapy, their effectiveness may be affected by a number of challenges and problems. Their use has been associated with both acquired and primary resistance (the tumor not responding to therapy when it is administered for the first time) [30]. Approximately one-third of patients show improvement during the first stage of treatment; however, resistance develops with time [31]. One of the main drawbacks of these medications is Immune-related Adverse Events (irAEs), which can occur early in therapy or later in treatment [32]. However, a recent review discussed the development of designer formulations in the pharmaceutical industry, highlighting their role in enhancing bioavailability, solubility, and stability. It examined the use of ionic liquids and deep eutectic solvents in improving drug permeability and solubilization [33]. These may assist in developing more precise ICIs with enhanced efficacy.
1.6. Adverse Effect Profile of ICIs
The chronic toxicities of these medicines, although frequently mild, can impact several organ systems and endure longer than anticipated. Fatal toxicities manifest in 0.4–1.2% of individuals, underscoring the necessity for additional research on long-term immunological consequences [33].
1.6.1. Immune-related Adverse Events to ICIs (irAEs)
These responses differ from the adverse effects caused by traditional chemotherapy. Many patients experience constitutional symptoms, skin rashes, and colitis [41]. Some individuals may even experience serious irAEs that negatively affect the liver, heart, endocrine glands, skin, and gastrointestinal tract [42, 43]. The specific drug being used and the patient's individual health circumstances dictate how often these adverse effects occur. ICIs have an anticipated 0.3 percent to 1.3 percent chance of a fatal side event [44]. Although the danger is still lower than with chemotherapy or stem cell transplantation, these lethal events usually happen early in the course of treatment [45, 46]. Additionally, the kind of side effect may differ based on the medications used in combination. For instance, patients on anti-CTLA-4 drugs have an increased risk of dying from colitis [47-49], but patients on anti-PD-1 or anti-PD-L1 drugs have an increased risk of dying from pneumonitis (Table 2) [44, 50, 51].
1.6.2. Cardiac Toxicity
There have been isolated reports of cardiotoxicity linked to ICIs; however, these cases are uncommon [57]. According to a recent report, patients treated aggressively for melanoma with ipilimumab and nivolumab experienced fulminant myocarditis, which unfortunately led to their deaths [57]. Interestingly, apart from hypertension, neither patient had any other significant risk factors. Eighteen occurrences (0.09%) of drug-related myocarditis were reported from a large database of 20,594 patients receiving ICIs. Remarkably, patients on combination therapy (ipilimumab plus nivolumab) had a higher incidence and severity of myocarditis (0.27% versus 0.06%) than individuals on nivolumab alone [59].
ICIs Group | Drug | Class | Approval Year | Indications/Refs. |
---|---|---|---|---|
CTLA-4 inhibitor | Ipilimumab 1,3,10 mg/kg IV |
IgG1mAb | 2010 | Melanoma [34] |
PD-1 inhibitor | Pembrolizumab 100,200,400 mg IV |
IgG4mAb | 2016 | GC, urothelial carcinoma, cervical cancer, melanoma, NSCLC, HNSCC, and TNBC [35] |
Nivolumab 120/240/480 mg IV |
2014 | Hodgkin's lymphoma, melanoma, NSCLC, RCC, HNSCC, HCC, ESCC, and pleural mesothelioma [36] | ||
Cemiplimab 350 mgIV |
2018 | Cutaneous squamous cell carcinoma [37] | ||
PD-LI inhibitor | Atezolizumab 840/1200/1680 mg IV |
IgG1mAb | 2016 | HCC, melanoma, SCLC, NSCLC, and urothelial carcinoma [38] |
Durvalumab 1500mg IV |
2017 | Urothelial bladder cancer, NSCLC, SCLC, BTC [39]. |
||
Avelumab 800 mgIV |
2017 | RCC, urothelial carcinoma, and Merkel cell carcinoma [40] |
ICI Group | Type of irAEs/ Refs. | Characteristics/ Refs. |
---|---|---|
Anti CTLA-4 antibodies |
Diarrhoea, Colitis, Hepatotoxicity Rashes, SJ Syndrome [52] Myocarditis Respiratory, Pneumonitis ITP [52], Endocrinopathy & Neuropathy [52] |
10-30%- serious irAEs [44], Dose-dependent Mostly occur within 8-12 weeks, Rashes- Earliest [53] Diarrhea, colitis- most common [44, 54-56] |
PD-1/PDL-1 inhibitors |
“Rash, fatigue, arthralgia, headache, pruritus Diarrhea, Colitis, Pneumonitis, hepatitis, and endocrinopathies [57] 2.9% of patients on anti-PD1 therapy- neurological” irAEs [58]. |
Fewer irAEs compared to anti-CTL4 antibodies [50] Common in the first 6 months [44] Skin, hepatic, and pulmonary-related irAEs are more frequent than anti-CTLA4 antibodies [44] |
1.6.3. Endocrine Adverse Drug Reactions
They can occur with ICI regimens, specifically causing inflammation of the pituitary gland, known as hypophysitis. This often leads to enlargement of the pituitary gland, with the anterior pituitary being more commonly affected than the posterior pituitary [60-63]. According to a meta-analysis by Barroso-Sousa et al 39% of patients with hypophysitis experienced symptoms that scored at least a grade 3 on the Common Terminology Criteria for Adverse Events [CTCAE] scale [63, 64]. Central hypothyroidism is the most common side effect of ICIs, followed by hypogonadism, and may be associated with central adrenal insufficiency, which may potentially lead to a fatal adrenal crisis. The occurrence of hypo physitis- hypo pituitarism induced by ipilimumab is not dependent on cumulative dosage or cycle frequency [62]. Nivolumab-ipilimumab combination therapy is associated with a higher incidence than with ipilimumab alone [64]. When using PD-1 inhibitors instead of ipilimumab, the frequency of these side effects is reduced, and the way these side effects manifest themselves also varies, indicating different pathways [65].
Thyroid dysfunction is another frequent adverse event that can manifest as either thyrotoxicosis or hypothyroidism, with hypothyroidism being the more common presentation [48]. Thyrotoxicosis is often caused by silent or destructive thyroiditis, followed by the development of hypothyroidism within 6 weeks [61, 66]. Although severe cases are uncommon and necessitate hospital admission, the majority of thyroid-related adverse events are either asymptomatic or produce mild to moderate symptoms [67]. Women in their later years may be more susceptible to thyroid dysfunction and may experience more severe symptoms of the condition [68-70]. The anti-PD-1 class of drugs has the largest risk of thyroid dysfunction, according to studies by Barbarossa-Sousa et al. [64]. On the other hand, the anti-CTLA-4 medication ipilimumab is linked to the least amount of thyroid impairment.
ICI treatment is linked to symptoms that resemble those of type 1 diabetes. These include high autoantibody levels and low or undetectable C-peptide levels [61, 70]. With ICI therapy, the incidence rates of type 1 diabetes mellitus vary from 0.2% to 0.9%. Rapid-onset diabetic ketoacidosis, on the other hand, has recently been linked to a more severe course of disease [71, 72]. Pembrolizumab and nivolumab had greater incidence rates than PD-L1 inhibitors [70, 73]. Additionally, combination therapy may raise the risk because symptoms may manifest sooner after therapy starts [70, 73].
Less than 2% of instances result in primary adrenal insufficiency, a rare symptom of irAE episodes. In a meta-analysis research by Barroso-Sousa et al., 43 cases of primary adrenal insufficiency were observed among 5,831 patients (0.7%), with 14 cases (0.3%) categorized as grade 3 or above [64].
1.6.4. Hematologic Adverse Drug Reactions
The ICI therapy can impact all hematopoietic cells, with the occurrence of high-grade anemia according to CTCAE ranging from 0.1% to 17%, neutropenia from 0.4% to 1.7%, and thrombocytopenia from 1.2% to 2.5% in the patients [74]. Other less common adverse reactions include hemolytic anemia, idiopathic thrombocytopenic purpura, bone marrow failure, and Hemophagocytic lymphohistiocytosis (HLH) [75, 76].
1.6.5. Skin-related Adverse Events
The most prevalent irCAEs involve lichenoid eruptions, vitiligo, pruritus, eczematous eruptions, psoriasiform eruptions, and medication eruptions associated with morbilliform disorders. Immunotherapy may be continued because they are usually not severe [77, 78]. The most frequent type of immune-related adverse event (irAE) is a morbilliform eruption, which is seen in twenty percent of patients taking PD-1/PD-L1 inhibitors and in half to two-thirds of patients receiving CTLA-4 inhibitors [79-81]. These eruptions, which manifest as itchy, consolidating macules and papules, can occur within the first 3-6 six weeks following the commencement of ICI.
Twenty-five to thirty-six percent of patients receiving CTLA-4 inhibitors and 47% of patients receiving combination ICI may experience pruritus, with or without rash [82, 83]. Nevertheless, refractory grade 3 pruritus affects less than 3% of patients [72, 74]. The onset often occurs during the first three to ten weeks of treatment [84].
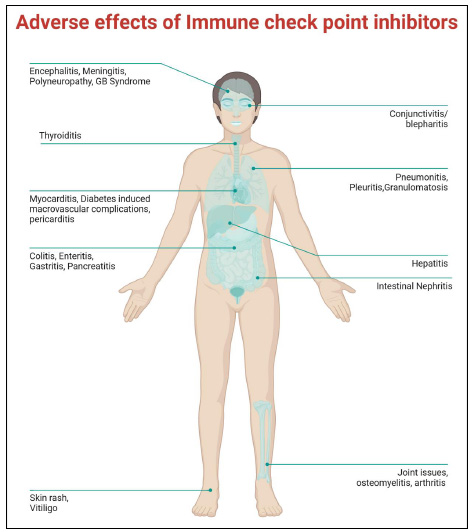
ICI treatment may sometimes lead to adverse outcomes. The figure represents organ-specific pathological outcomes in response to ICI therapy.
Cancer Type | Approved ICIs | Clinical Outcomes | Key References |
---|---|---|---|
Melanoma | Pembrolizumab, Nivolumab, Ipilimumab | Improved overall survival (OS) & progression-free survival (PFS) | KEYNOTE-006, CheckMate-067 |
Non-Small Cell Lung Cancer (NSCLC) | Pembrolizumab, Nivolumab, Atezolizumab, Durvalumab | Prolonged OS, durable responses, superior to chemotherapy | KEYNOTE-024, PACIFIC Trial |
Renal Cell Carcinoma (RCC) | Nivolumab, Atezolizumab | Increased OS & objective response rates (ORR) | CheckMate-214, JAVELIN Renal 101 |
Hepatocellular Carcinoma (HCC) | Nivolumab, Pembrolizumab, Atezolizumab + Bevacizumab | Improved PFS & OS, particularly in combination therapy | IMbrave150, KEYNOTE-240 |
Head and Neck Squamous Cell Carcinoma (HNSCC) | Nivolumab, Pembrolizumab | Prolonged OS in recurrent/metastatic cases | KEYNOTE-048, CheckMate-141 |
Triple-Negative Breast Cancer (TNBC) | Atezolizumab, Pembrolizumab | Improved PFS in PD-L1+ tumors | IMpassion130, KEYNOTE-355 |
Colorectal Cancer (MSI-H/dMMR) | Pembrolizumab, Nivolumab | High ORR & durable responses in MSI-H patients | KEYNOTE-177, CheckMate-142 |
Bladder Cancer (Urothelial Carcinoma) | Atezolizumab, Durvalumab, Nivolumab, Pembrolizumab | Improved OS & durable responses in platinum-refractory cases | IMvigor210, KEYNOTE-045 |
Hodgkin’s Lymphoma | Nivolumab, Pembrolizumab | High ORR & PFS in relapsed/refractory cases | CheckMate-205, KEYNOTE-087 |
Eczematous dermatitis affects up to 68% of patients on anti-CTLA-4 agents and about twenty percent of patients on PD-1/PD-L1 inhibitors, with an early onset occurring within 6 weeks of therapy [84]. Clinical symptoms include papules and poorly defined, coalescing erythematous patches with flexural predominance and subsequent skin changes [81]. Vitiligo is a common skin manifestation in patients receiving ICIs for metastatic melanoma, often accompanied by poliosis [77, 79, 84]. Another skin symptom that affects 1-5% of patients on PD-1/PD-L1 inhibitors is bullous pemphigoid (BP) [84]. Due to its delayed onset, BP is one of the irCAEs with longer latencies [77, 83, 85].
In rare cases, although with a poor prognosis (10% for SJS and 50% for TEN), PD-1/PD-L1 inhibitors and CTLA-4 can result in Severe Cutaneous Adverse Reaction (SCAR), such as SJS and TEN [83]. Constitutional symptoms and generalized erythema are the initial signs of these responses. Flaccid bullae with epidermal separation and mucosal involvement affecting different body areas follow [83]. Morbilliform eruptions in patients using PD-1/PDL-1 inhibitors should be regularly examined as they may suggest progression to a SCAR [81, 85, 86]. A schematic representation is provided summarizing all the adverse outcomes in response to ICI dosage (Fig. 1).
Further, the individuals on treatment with ICIs are known to become resistant by virtue of various mechanisms. The treatment of Non-Small Cell Lung Cancer (NSCLC) has been revolutionized by Immune Checkpoint Inhibitors (ICIs), which have expanded the treatment of the disease from advanced to early stages. Nevertheless, Immunotherapy (IO) resistance continues to be a challenge, which is motivating research into resistance mechanisms and novel therapeutics that target the Tumor Microenvironment (TME) and immune checkpoints for precision immuno-oncology [87].
Cytotoxic T-lymphocyte-associated protein 4 (CTLA-4) and the programmed cell death protein 1 (PD-1)/programmed death-ligand 1 (PD-L1) axis are the targets of immune checkpoint inhibitors that have revolutionized oncology by improving anti-tumor immune responses. Resistance—both primary and acquired—continues to pose a challenge despite the fact that some patients achieve durable responses. Development of predictive biomarkers and optimization of treatment strategies may be facilitated by an understanding of the molecular and immunologic mechanisms of ICI response and resistance [88]. The Tumor Immune Microenvironment (TME) was analyzed to investigate immune checkpoint inhibitor resistance in advanced non-small cell lung cancer (NSCLC). The necessity of personalized treatment strategies was underscored by the association between primary resistance and poor CD8+ T cell infiltration, while acquired resistance was associated with myeloid-derived suppressor cells, M2 macrophages, reduced tumor-reactive CD8+ T cells, or decreased neoantigen presentation [89]. Henceforth, understanding these mechanisms will help in identifying the potential targets. Further, understanding the long-term effect of the ICIs in various tumours will be of vital interest.
1.7. Case Studies Demonstrating the use of ICIs in Various Cancers
A patient with lung squamous cell carcinoma and lymphoma, previously treated with chemoradiotherapy and a new PD-1 monoclonal antibody, experienced severe Checkpoint Inhibitor Pneumonitis (CIP) and was brought to the ICU due to respiratory failure. Intensive care physicians administered anti-infective medication, with hydration management, corticosteroids, and respiratory and nutritional support for the case. Metagenomic next-generation sequencing (mNGS) excluded severe infections, facilitating tailored CIP therapy. The patient's condition stabilized, resulting in a successful discharge. This instance highlights the necessity for early detection of CIP, with mNGS facilitating its differentiation from severe infections [90]. The 54-year-old lady with Stage IIIb squamous cell carcinoma (cT2aN3M0) had her first chemoradiotherapy. After two years, drug-induced pneumonitis led to the discontinuation of nivolumab treatment due to cancer recurrence. Prednisolone and eight cytotoxic medicines did not stop cervical lymph node metastases.
The tumor expressed 10% PD-L1. She underwent palliative neck radiotherapy for enlarged lymph nodes after a ninth-line regimen of cisplatin, gemcitabine, and necitumumab four years after diagnosis. After three months of atezolizumab as the tenth-line treatment, tumor reduction was considerable, suggesting radiation and cisplatin may have raised PD-L1 expression. This highlights the need for more studies on treatment history and PD-L1 expression in squamous cell carcinoma [91].
Following treatment with atezolizumab and tiragolumab, a 64-year-old male with laryngeal squamous cell carcinoma developed immune-mediated hepatitis, which ultimately resulted in liver failure. A complete recovery was achieved through intensive care management and plasma exchange [92]. A 79-year-old man with metastatic renal clear cell carcinoma who was receiving nivolumab developed dysphagia and choledocholithiasis. Endoscopy demonstrated esophagitis, with histopathology results suggesting nivolumab-associated lymphocytic esophagitis. Proton pump inhibitors and steroids were administered to manage the condition [93].
The CheckMate 141 and KEYNOTE 040 trials demonstrated that anti-PD1 monotherapy improved overall survival in patients with recurrent or metastatic head and neck squamous cell carcinoma who had failed platinum-based therapy.
Standard chemotherapy was outperformed by both nivolumab and pembrolizumab [94]. Despite the fact that immune checkpoint inhibitors (ICI) can cause side effects, the CheckMate 141 and KEYNOTE 040 trials have demonstrated their advantages, which will enable the development of precision-based strategies to reduce off-target effects. A table representing the various cancer-specific ICIs is represented (Table 3).
1.8. The Role of Artificial Intelligence in Machine Learning for Predicting the Outcome of ICI Treatment
Few studies have demonstrated the potential benefits of artificial intelligence and machine learning models in the context of ICIs. For example, a deep learning model, Deep-IO, was created to predict the ICI response in NSCLC patients using H&E images. This model outperformed TMB and PD-L1 expression. The predictive accuracy of Deep-IO was substantially enhanced by the combination of PD-L1 scores, underscoring its potential for optimizing immunotherapy selection [95]. LORIS, a six-feature logistic regression model, was engineered to outperform current biomarkers in the prediction of Immune Checkpoint Blockade (ICB) response across 18 solid tumors. It provided a precise tool for optimizing immunotherapy decisions by accurately stratifying patients based on response probability and survival [96]. Advancements in AI and machine learning have enabled predictive modeling of PD-L1, TMB, and TME, improving immunotherapy selection in lung cancer. Integrating multi-omics data with AI-driven “digital biopsy” may enhance precision medicine and clinical decision-making [97]. To summarize, AI and ML have opened new avenues to understand the various aspects of ICIs.
CONCLUSION
Our understanding of the safety and efficacy of immune checkpoint inhibitors (ICIs) has significantly improved over the past decade, providing valuable insights into their therapeutic potential. The utilization of ICIs in cancer treatment has significantly improved patient outcomes; however, they are not universally efficacious and present unique obstacles. A significant proportion of patients either do not respond to ICIs (primary resistance) or eventually develop resistance (acquired resistance), which restricts their long-term efficacy. This is a significant limitation. Furthermore, Immune-related Adverse Events (irAEs) are frequently associated with ICIs. These events can be moderate to life-threatening and frequently necessitate immunosuppressive management, which has the potential to compromise the anti-tumor response.
Artificial Intelligence (AI) and Machine Learning (ML) have begun to substantially improve our methodology regarding ICIs, in addition to therapeutic improvements. In order to discover new biomarkers, enhance treatment protocols, and forecast responses to ICIs, AI-driven models can evaluate extensive patient data. In addition, the early detection of irAEs can be enhanced by machine learning algorithms, which can facilitate prompt intervention and help to enhance patient safety. Nevertheless, the accuracy and generalizability of AI and ML-based models may be influenced by the current limitations in data availability and standardization, which are dependent on high-quality and diverse datasets.
The incorporation of ICIs with chemotherapy, targeted treatments, and radiotherapy has expanded their application beyond monotherapy, leading to enhanced clinical outcomes. The development of predictive biomarkers, such as PD-L1 expression and Tumor Mutational Burden (TMB), has improved patient selection, ensuring that ICIs are administered to the individuals who are most likely to benefit from them. Nevertheless, these biomarkers are not always dependable, as some patients with high PD-L1 expression or TMB still fail to respond, while others with low levels may derive benefit. This underscores the necessity of more accurate predictive tools.
Although advancements have been made, there are still obstacles to overcome in the areas of optimizing patient selection, managing resistance, mitigating toxicities, and addressing high treatment costs. It is imperative to exercise caution when selecting appropriate ICIs, whether as monotherapy or in combination with other therapies, in order to optimize their clinical efficacy in cancer treatment. The integration of AI and ML into this dynamic environment enhances our ability to personalize treatments and enhance patient outcomes; however, additional research is required to further refine their clinical utility.
AUTHORS’ CONTRIBUTIONS
The authors confirm their contribution to the paper as follows: SA and AKB contributed to writing, reviewing, and editing, while SV was responsible for the conceptualization. All authors reviewed the results and approved the final version of the manuscript.
LIST OF ABBREVIATIONS
AI | = Artificial Intelligence |
AML | = Acute Myeloid Leukemia |
BTC | = Biliary Tract Cancer |
CIP | = Checkpoint Inhibitor Pneumonitis |
CTLA-4 | = Cytotoxic T-Lymphocyte-Associated Protein 4 |
CTCAE | = Common Terminology Criteria for Adverse Events |
FDA | = Food and Drug Administration |
GC | = Gastric Cancer |
HCC | = Hepatocellular Carcinoma |
H&E | = Hematoxylin and Eosin |
HNSCC | = Head and Neck Squamous Cell Carcinoma |
HLH | = Hemophagocytic Lymphohistiocytosis |
ICIs | = Immune Checkpoint Inhibitors |
ITP | = Idiopathic Thrombocytopenic Purpura |
irAE | = Immune-related Adverse Event |
IO | = Immuno-Oncology |
mAb | = Monoclonal Antibody |
ML | = Machine Learning |
mNGS | = Metagenomic Next-Generation Sequencing |
MSI | = Microsatellite Instability |
NSCLC | = Non-Small Cell Lung Cancer |
ORR | = Objective Response Rate |
OS | = Overall Survival |
PD-1 | = Programmed Cell Death Protein 1 |
PD-L1 | = Programmed Death-Ligand 1 |
PD-L2 | = Programmed Death-Ligand 2 |
PFS | = Progression-Free Survival |
RCC | = Renal Cell Carcinoma |
SCAR | = Severe Cutaneous Adverse Reaction |
SJS | = Stevens–Johnson Syndrome |
TEN | = Toxic Epidermal Necrolysis |
TMB | = Tumor Mutational Burden |
TME | = Tumor Microenvironment |
TNBC | = Triple-Negative Breast Cancer |
CONFLICT OF INTEREST
Dr. Sampara Vasishta is the Editorial Advisory Board member of the journal The Open Biomedical Engineering Journal.
ACKNOWLEDGEMENTS
Authors thank Central Research Laboratory for Molecular Genetics, Bioinformatics and Machine Learning at Apollo Institute of Medical Sciences and Research Chittoor, for providing the infrastructure for this study.